Method development for electron dynamics
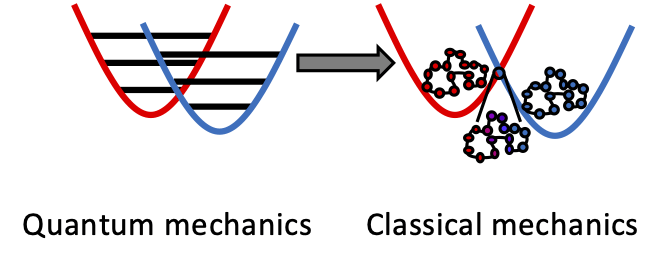
A large focus of the Kretchmer group is on the development of new methods for the direct simulation of electron dynamics. We work with a variety of different methods including classically isomorphic and real-time electronic structure methods. In the former, a quantum mechanical system is mapped to a classical system, such that the underlying quantum dynamics can be treated using purely classical equations of motion. In the latter, we take ideas from static electronic structure theory to solve the time-dependent Schrodinger equation.
Charge transport in novel material systems
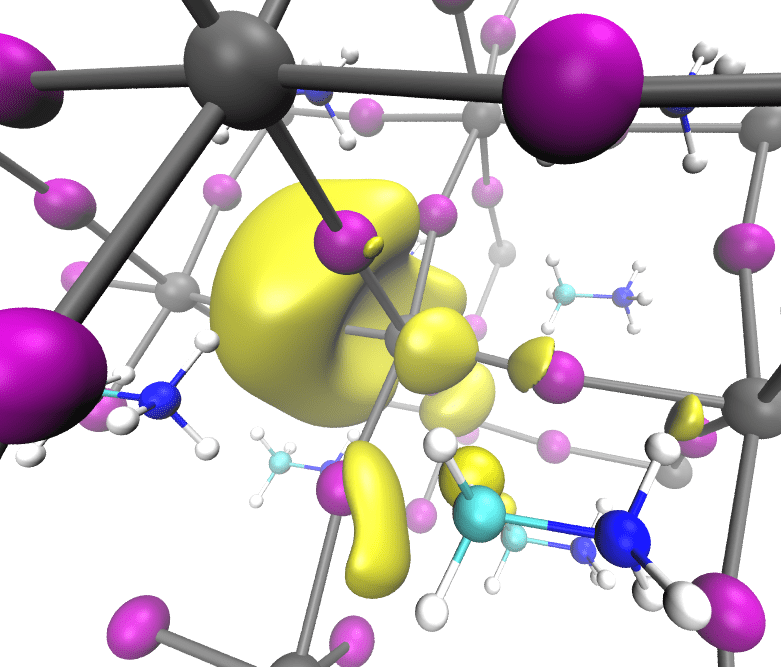
We are actively exploring charge and spin transport processes in new material systems exhibiting novel photochemical properties. In many cases, conventional theories are insufficient to describe the charge transport mechanism, due to the unique behavior of the surrounding nuclear or electronic environment. To tackle this lack of understanding, we utilize a multi-tiered simulation approach consisting of rare-event sampling techniques, path integral molecular dynamics, and innovative real-time electronic structure methods. Current interests include 3D and 2D perovskites as well as chiral-induced spin selectivity.
Real-time dynamics of ultrafast electronic decay mechanisms
The ionization of an inner valence or core electron can initiate competing electronic relaxation pathways that occur on the attosecond to femtosecond timescale. These include interatomic coulomb decay, in which an electron relaxes to fill the hole while simultaneously ionizing another electron on a neighboring molecule, and electron transfer mediated decay, in which an electron transfers from a neighboring molecule to fill the hole simultaneously ionizing another electron, among others. We develop and apply real-time DFT methods to directly simulate the real-time dynamics of these competing electronic decay mechanisms in molecular clusters and at surfaces.
Non-adiabatic dynamics in the presence of a quantized electric field

Many exciting features emerge when the interaction of light with matter is treated quantum mechanically. In the weak-coupling regime, the quantum nature of light leads to fluorescence processes. While in the strong-coupling regime, as is present in optical cavities or plasmonic arrays, new hybridized quantum states are formed comprised of mixtures of the quantum states of light and matter. This mixing can significantly alter the efficiency and outcome of chemical processes. We are developing new quantum-dynamical methods that can readily be combined with classical sampling techniques to directly simulate the non-adiabatic dynamics of chemical systems coupled to quantum light.